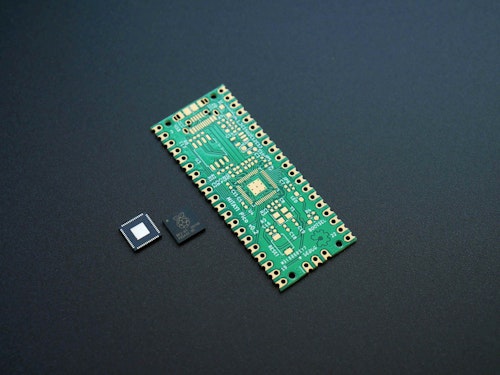
Frequency Domain Reflectometers (FDR): How They Work and Why They Matter
Introduction to Frequency Domain Reflectometer (FDR)
Optical reflectometry is crucial in diagnosing and characterizing optical networks, components, and materials. Among the various techniques available, Frequency-Domain Reflectometry (FDR) stands out for its high-resolution measurements and ability to precisely analyze short-range optical systems. Unlike traditional Time-Domain Reflectometry (TDR) methods, which rely on pulse timing, FDR leverages frequency variations to detect faults, measure insertion loss, and assess material properties with greater sensitivity.
This article explores the fundamentals of FDR, its applications in fiber optic sensing and material characterization, and how Santec’s advanced Optical Frequency Domain Reflectometers push the boundaries of optical testing.
What is a Frequency-Domain Reflectometer (FDR)?
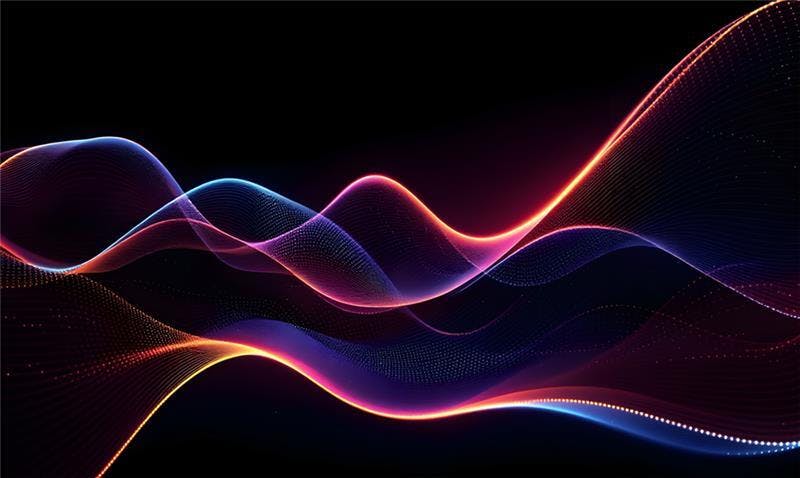
A Frequency-Domain Reflectometer (FDR) is a specialized instrument designed to detect faults and analyze optical and electrical systems with high precision. By transmitting a range of frequencies into a medium and examining how different frequencies reflect, FDR provides a detailed profile of impedance variations, structural changes, or defects.
Unlike Time-Domain Reflectometry (TDR), which measures signal reflection based on time delay, FDR leverages frequency-domain analysis to extract high-resolution spatial information. This approach makes it particularly effective for short-range, high-precision measurements in applications such as optical fiber diagnostics, material characterization, and circuit testing.
This instrument is widely used in fiber optic monitoring, electrical component testing, and material characterization applications. It provides high-resolution fault detection and precise measurements of impedance variations.
Key Components of an FDR Instrument
An FDR system consists of three main components:
● Signal Source: The signal source generates a continuous wave (CW) signal that sweeps across various frequencies. This ensures a broad-spectrum analysis of reflections from different parts of the system.
● Analyzer: The analyzer processes the reflected signals and measures the variations in amplitude and phase at different frequencies. Capturing these responses determines the locations and characteristics of faults or discontinuities.
● Detector: The detector converts the measured frequency data into a readable format, often using Fast Fourier Transform (FFT) or Inverse FFT to map frequency variations into spatial or material properties.
Operational Concept of Frequency-Domain Analysis
FDR operates by injecting a signal with a sweeping frequency range into the system under test. As the signal encounters changes in impedance—caused by defects, discontinuities, or variations in material properties—part of it is reflected back. The instrument measures the reflected signal at each frequency, building a frequency-dependent system profile.
By applying mathematical transformations, such as Inverse Fast Fourier Transform (IFFT), FDR converts these frequency-domain data points into a spatial representation, allowing users to precisely locate faults or analyze material characteristics. This method provides exceptional resolution in short-range measurements, making it particularly useful in laboratory and controlled environments requiring high precision.
Uses of Frequency-Domain Reflectometer (FDR)
Optical Fiber Sensing
One of the primary applications of frequency-domain reflectometry is in distributed fiber optic sensing, which is crucial in real-time monitoring of environmental conditions along optical fibers.
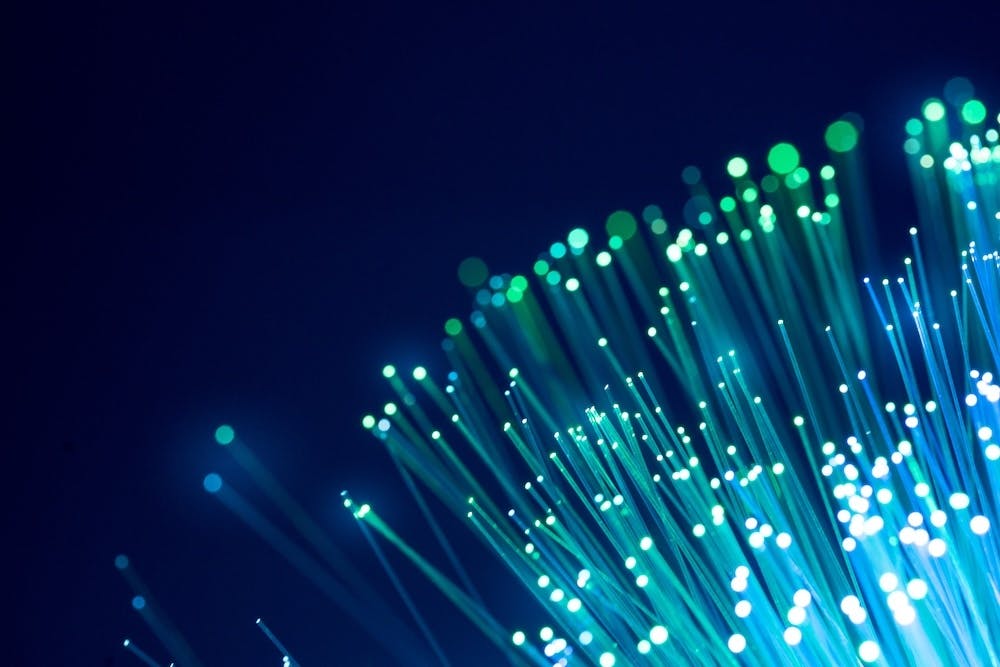
How FDR is Used for Distributed Sensing
FDR enables continuous monitoring of optical fibers by detecting subtle changes in their physical properties. Instead of requiring discrete sensors placed at fixed points, FDR allows for a distributed approach, where the fiber acts as a sensor. This is achieved by analyzing how different frequency components interact with the fiber’s material and identifying variations in its response.
Measuring Temperature, Strain, and Vibration
Analyzing phase shifts and frequency variations helps FDR detect changes in:
● Temperature: Thermal expansion and refractive index changes within the fiber affect the reflected signal’s frequency response.
● Strain: Mechanical stress on the fiber alters its optical properties, which can be detected using frequency analysis.
● Vibration: Periodic disturbances cause distinct frequency shifts, allowing FDR to monitor vibrations with high sensitivity.
Real-World Applications in Telecommunications and Infrastructure Monitoring
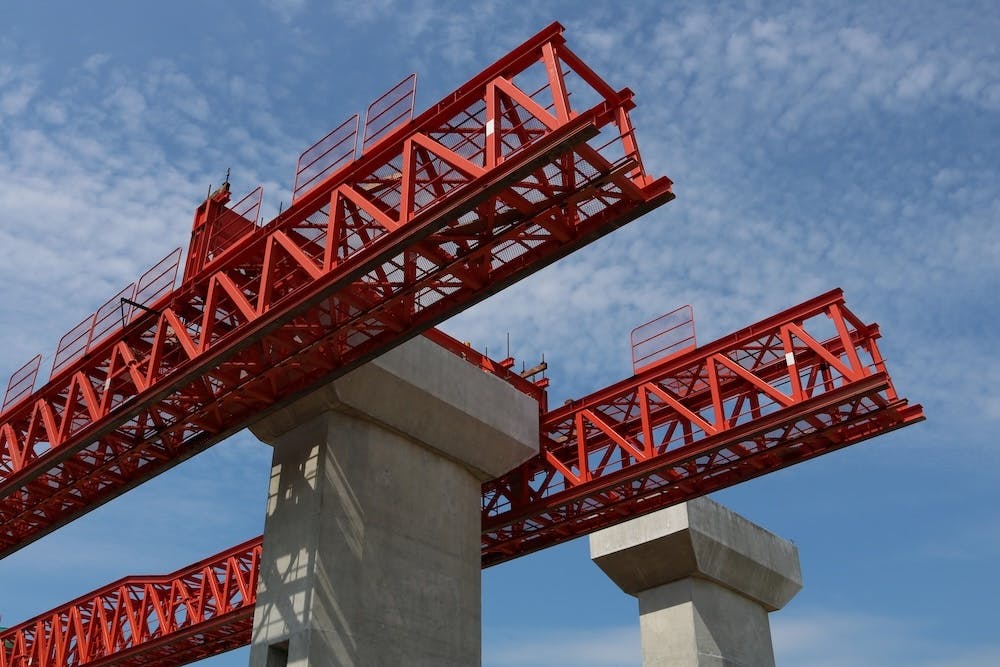
FDR-based fiber sensing is widely used in:
● Telecommunications networks detect fiber faults, microbends, or signal degradation before they cause failures.
● Structural health monitoring of bridges, tunnels, and pipelines, where continuous strain measurements help predict potential failures.
● Energy sector applications, such as monitoring high-voltage power lines, where optical fibers are embedded to track environmental stresses.
This ability to provide real-time, distributed sensing makes FDR an indispensable tool for industries requiring precise condition monitoring over long distances.
Material Characterization
Beyond fiber optics, frequency-domain reflectometry is key in material analysis, particularly in evaluating thin films, coatings, and composite materials.
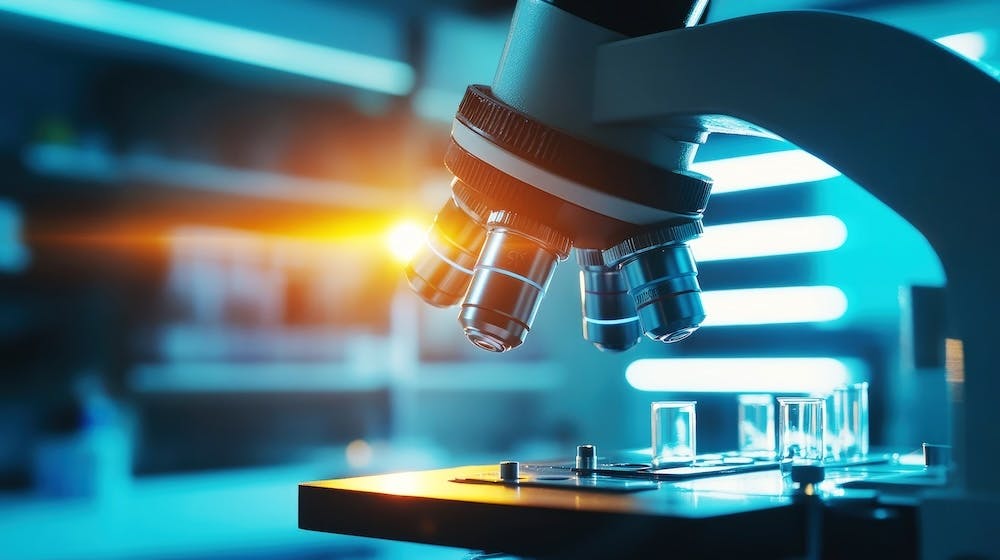
How FDR is Used to Analyze Thin Films and Coatings
In material science, thin films and coatings often require non-destructive testing methods to determine their properties. FDR analyzes these materials by measuring how different frequencies interact with their surface and internal structure.
By examining how signals reflect at varying frequencies, researchers can extract valuable information such as:
● Thickness: The interference patterns of reflected signals indicate the exact thickness of coatings.
● Dielectric properties: Changes in impedance response help determine permittivity and conductivity.
● Surface uniformity: Variations in reflection reveal inconsistencies or defects in coatings.
Importance in Semiconductor Manufacturing and Material Research
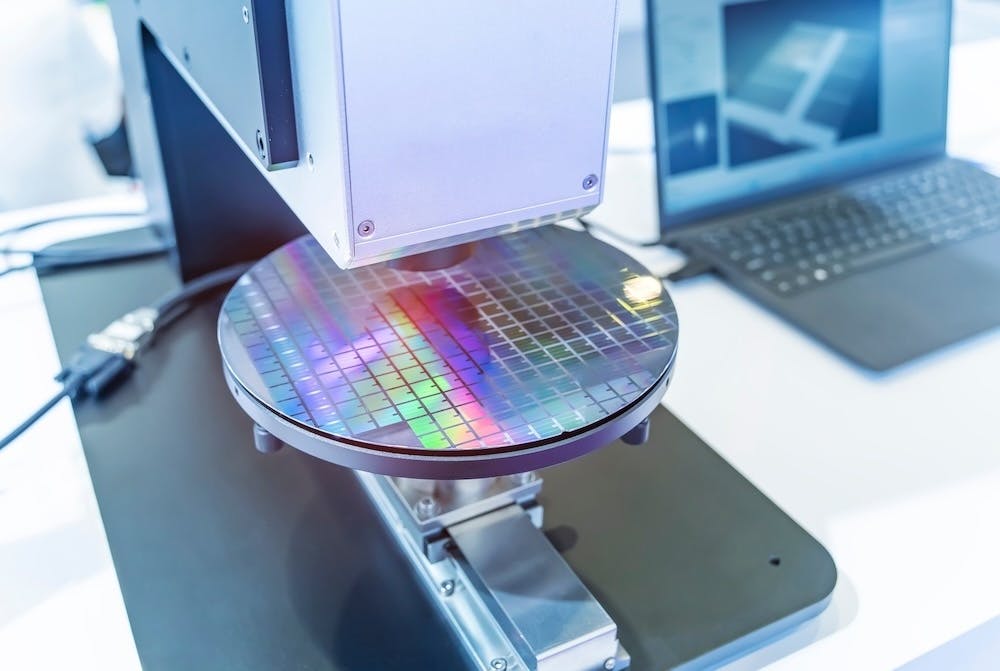
FDR is widely used in:
● Semiconductor fabrication, where precision measurement of thin-film layers ensures optimal performance of microelectronic devices.
● Aerospace and automotive industries, where material coatings must meet stringent quality standards for durability and performance.
● Biomedical research, where FDR is used to analyze biomaterial coatings in medical devices.
Advantages Of Traditional Material Characterization Techniques
Compared to conventional methods like optical microscopy or mechanical thickness measurement, FDR provides several advantages:
● Non-destructive testing ensures materials remain intact while being analyzed.
● High sensitivity and precision allow for detecting microscopic variations.
● Faster analysis times reduce the need for labor-intensive testing procedures.
With its ability to analyze material properties with high accuracy, FDR is revolutionizing material characterization, particularly in industries requiring precise quality control and advanced research applications.
Comparing Optical Frequency Domain Reflectometry (OFDR) and Optical Time Domain Reflectometry (OTDR)
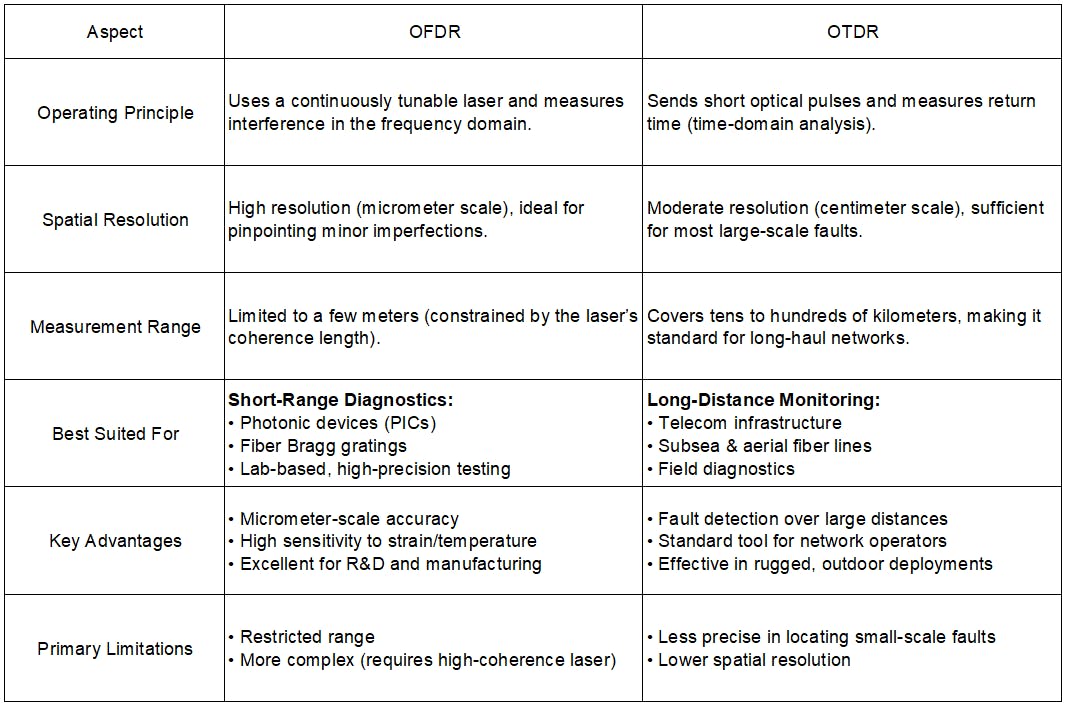
Operating Principle
Optical Frequency Domain Reflectometry (OFDR)
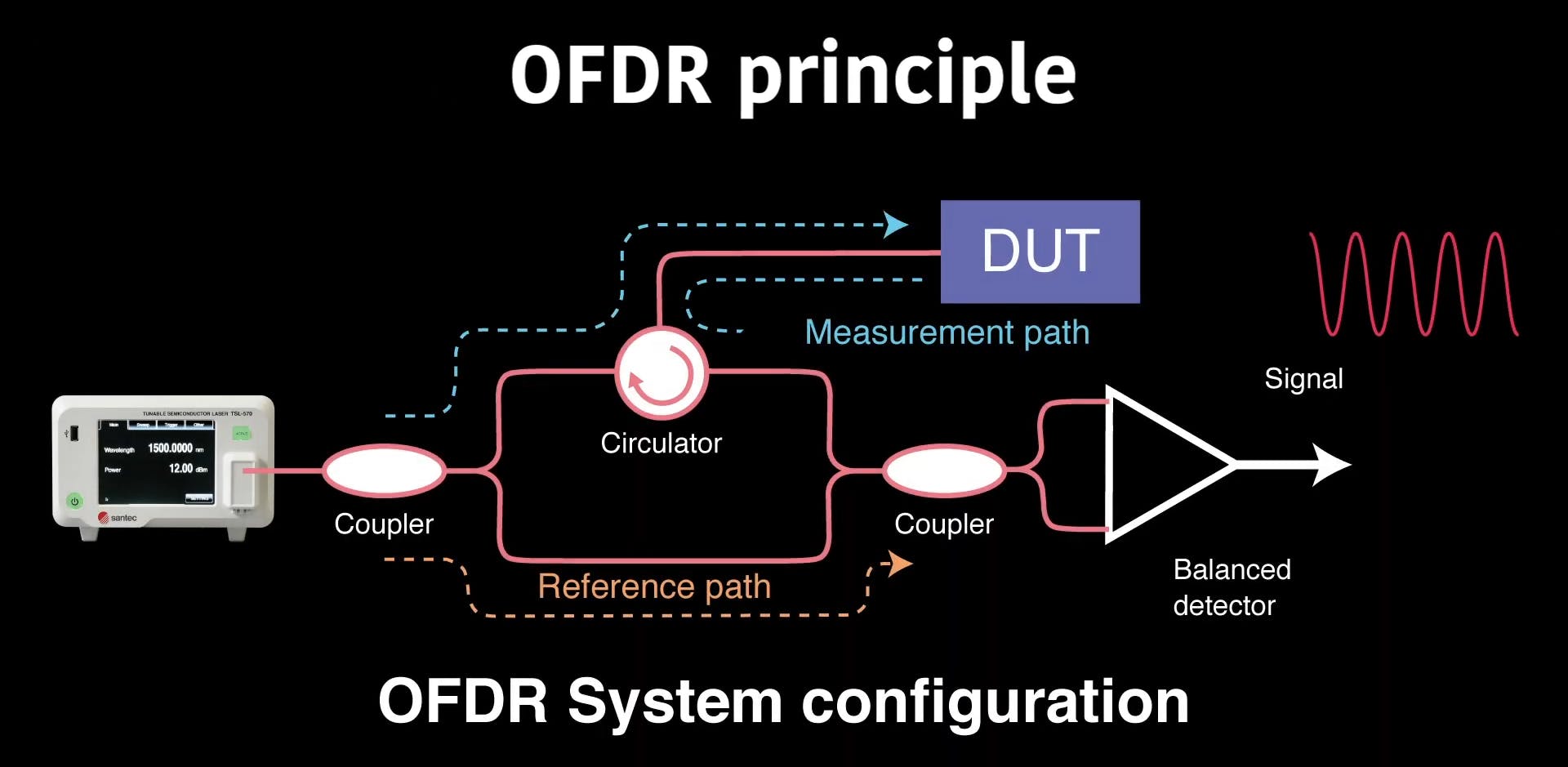
Optical Frequency Domain Reflectometry (OFDR) is an advanced technique that analyzes optical fiber properties using frequency-domain analysis. Instead of sending time-based pulses, OFDR utilizes a continuously tunable laser to sweep across a range of optical frequencies. The system then measures the interference between the reference signal and the backscattered light from the fiber under test.
This technique relies on high-coherence lasers and Fourier transform calculations to map reflections within the fiber. By processing the interference signals mathematically, OFDR constructs a detailed spatial representation of the fiber or optical component.
Benefits in High-Resolution, Short-Range Measurements
● OFDR achieves exceptional spatial resolution, often down to the micrometer scale, making it ideal for detecting minor variations within short optical fibers.
● It is well-suited for component-level testing, such as analyzing photonic integrated circuits (PICs) and compact optical assemblies.
● OFDR enables detailed fiber strain, temperature, and birefringence profiling, making it indispensable in precision research and manufacturing environments.
While OFDR delivers high accuracy, its measurement range is limited due to the coherence length of the laser source, restricting it to short-range applications.
Optical Time Domain Reflectometry (OTDR)
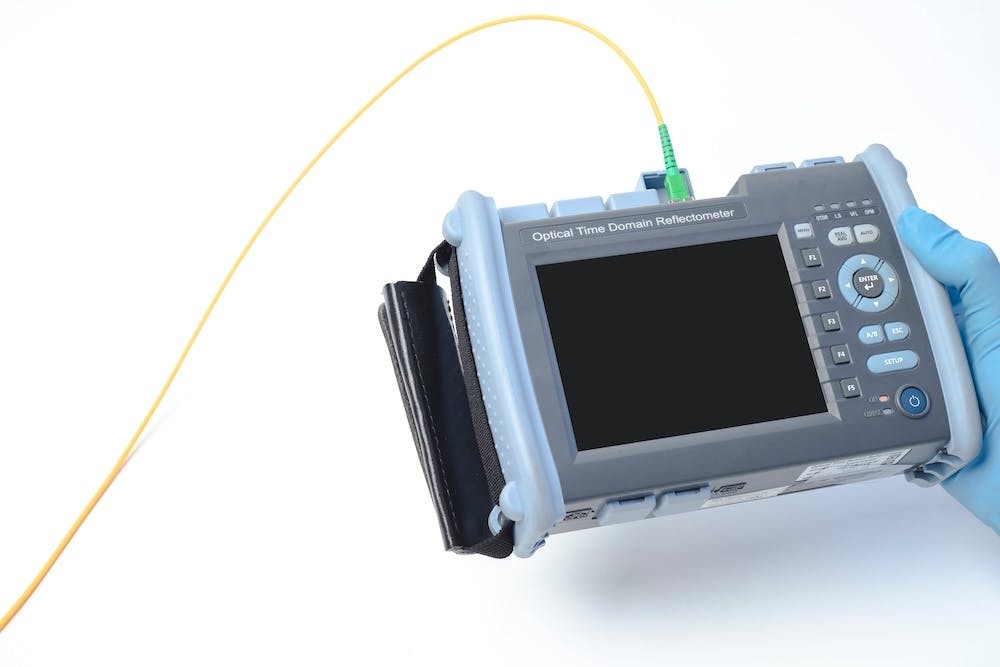
Optical Time Domain Reflectometry (OTDR) operates on a time-domain analysis principle, utilizing short optical pulses to detect and locate discontinuities in fiber optic networks. Instead of sweeping through different frequencies like Optical Frequency Domain Reflectometry (OFDR), OTDR injects controlled pulses of light into the fiber and measures the time it takes for reflections to return.
The system records both Rayleigh backscattering and discrete reflections caused by splices, breaks, or other discontinuities. By analyzing the backscattered signal over time, OTDR converts this data into distance measurements using the known speed of light in the fiber. This approach makes OTDR essential for long-distance fault detection, particularly in telecommunications, data centers, and large-scale fiber optic networks.
Application in Long-Distance Optical Fiber Fault Detection
● OTDR is highly effective for monitoring telecommunication networks, where detecting fiber breaks and signal losses across kilometers is critical.
● It is used in outside plant (OSP) installations, where engineers must identify cable faults in large-scale fiber deployments.
● The technique is also valuable in subsea and aerial fiber networks, ensuring signal integrity in critical infrastructure.
While OTDR lacks the satisfactory spatial resolution of OFDR, its ability to scan tens or even hundreds of kilometers makes it an essential tool for long-haul fiber diagnostics.
Resolution and Precision
OFDR: High Precision for Short-Distance Optical Testing
OFDR is recognized for its unparalleled spatial resolution, often in the micrometer range. This level of precision is achieved by analyzing the phase and amplitude changes in the frequency spectrum rather than relying on time delay measurements.
Why OFDR is Preferred for Short-Distance, High-Precision Applications
● Micrometer-scale accuracy enables precise analysis of compact optical components, ensuring high-resolution defect detection and performance evaluation.
● Ideal for photonic device testing, including waveguides, fiber Bragg gratings (FBGs), and optical sensors, where precision is critical.
● Non-intrusive and high-sensitivity measurements allow for detailed strain and temperature mapping in fibers, enhancing diagnostics in optical networks.
● Proximity sensing for automated wafer-level testing enables precise, contactless characterization of photonic integrated circuits (PICs) and semiconductor wafers, ensuring quality control in high-volume manufacturing environments.
Since OFDR provides continuous, high-resolution data, it is preferred in controlled environments where small-scale diagnostics are required, such as research labs and manufacturing facilities.
OTDR: Effective for Long-Range Diagnostics
Compared to OFDR, OTDR has a lower spatial resolution, often in centimeters rather than micrometers. However, this trade-off is acceptable in applications where long-distance monitoring is more critical than pinpoint precision.
Situations Where OTDR is Preferable Despite Lower Precision
● Long-haul fiber network maintenance, where detecting faults over large distances is essential.
● Telecommunication backbone monitoring, where the goal is to ensure signal integrity rather than analyzing minor imperfections.
● Field deployments require rugged, portable instruments to inspect optical networks across cities and regions.
Although OTDR does not provide the fine resolution of OFDR, its ability to detect issues over tens of kilometers makes it a practical and essential tool for fiber optic network operators.
Distance Range
OFDR: Limited Range Due to Coherence Constraints
One of OFDR’s primary limitations is its measurement range, which is constrained by the coherence length of the laser source. Coherence length defines the distance over which the laser maintains a stable phase relationship, and in OFDR, this typically restricts measurements to a few meters.
Why OFDR Excels in Lab-Based and Component-Level Testing
• Ideal for short-range applications, including detailed analysis of optical sensors, photonic integrated circuits (PICs), and other high-precision components.
• The high spatial resolution makes it a top choice for fiber characterization in controlled environments and inline quality control in production settings.
• Applicable in automated wafer-level and component testing, particularly in proximity sensing for probe stations, where non-contact measurement ensures high accuracy and efficiency in manufacturing lines.
• Useful for structural health monitoring in small-scale sensing applications, where pinpoint accuracy is crucial for detecting micro-strain and temperature variations.
While OFDR’s range is limited, its high precision and automation capabilities make it highly valuable for R&D environments and high-throughput production testing.
OTDR: Long-Distance Fault Detection in Optical Fiber Networks
Unlike OFDR, OTDR can analyze optical fibers over tens or hundreds of kilometers. This makes it the industry standard for large-scale fiber optic network diagnostics.
Importance in Large-Scale Fiber Optic Networks
● Essential for telecom infrastructure, where fiber spans across cities and countries.
● Used in subsea fiber cables, where detecting faults remotely is critical for maintenance teams.
● Deployed in data centers and metro networks, ensuring stable connections between nodes.
The ability to monitor fiber health over long distances makes OTDR indispensable for network reliability and maintenance, even if it lacks the satisfactory resolution of OFDR.
Santec’s Optical Frequency Domain Reflectometers
Swept Photonics Analyzer (SPA) Series
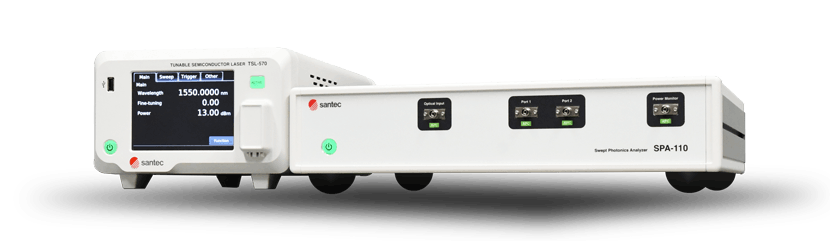
Swept Photonics Analyzer
Santec’s Swept Photonics Analyzer (SPA) series represents a significant advancement in optical testing, offering high-precision measurement capabilities for various optical components. The series includes models such as the SPA-110, each designed to meet specific testing requirements.
The SPA-110 extends the measurement range from 5 meters to 30 meters. This enhancement accommodates additional optical signal conditioning devices, such as polarization controllers and optical switches, within the testing setup. The extended range of SPA-110 truly shines in its ability to thoroughly characterize small devices like silicon photonic circuits, fiber optic components, and compact fiber optic assemblies.
The SPA-110 is available in O-band (1260 nm to 1350 nm) and CL-band (1480 nm to 1640 nm) configurations, providing flexibility to match the specific wavelength requirements of the devices under test.
Swept Photonics Analyzer (Long Range Model)
The SPA-110, as the long-range model in the SPA series, offers an extended measurement range of up to 30 meters. This capability is particularly beneficial for analyzing longer fiber optic cable assemblies with closely spaced components, where traditional Optical Time Domain Reflectometry (OTDR) technology might struggle to distinguish between them.
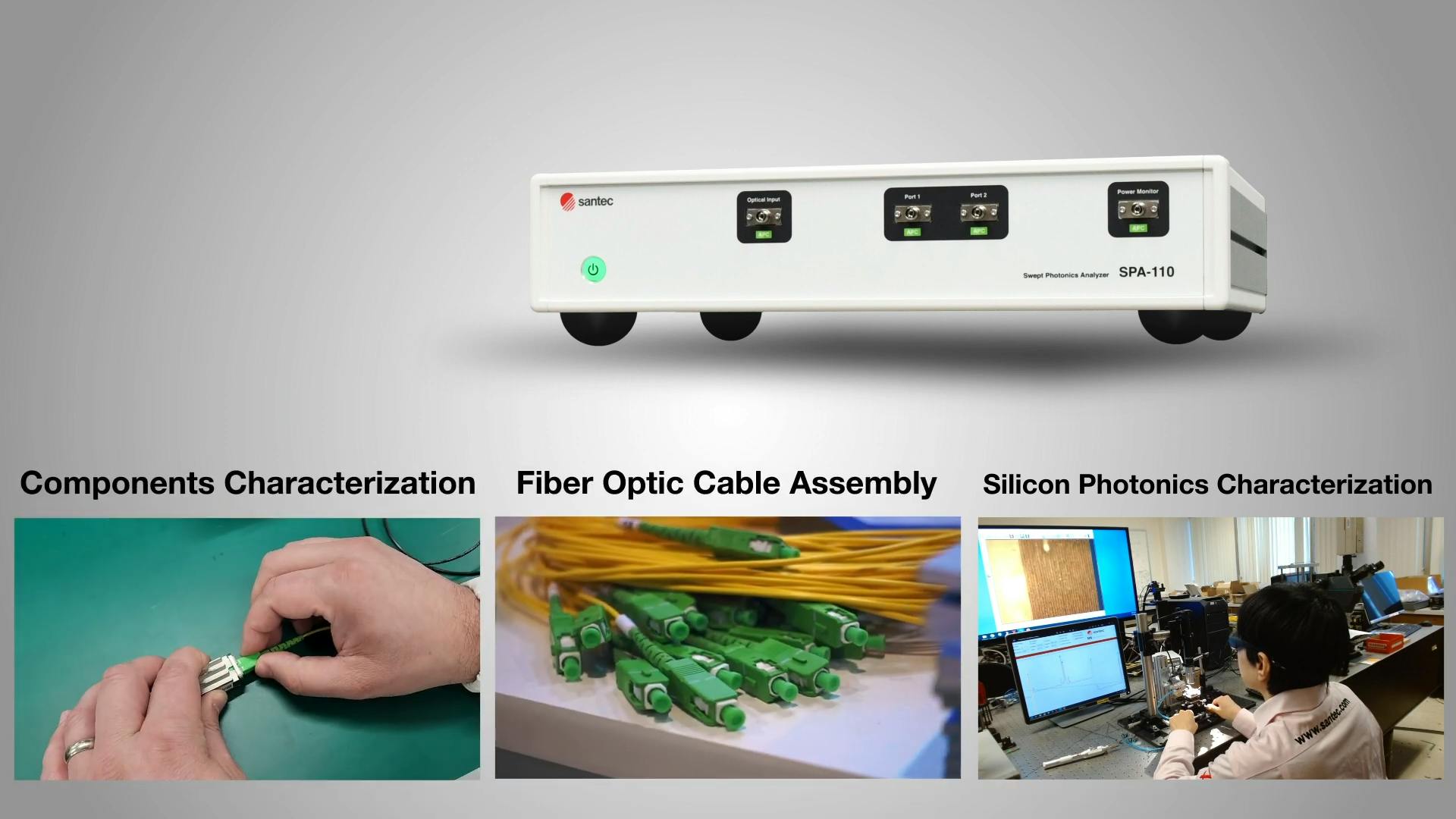
The extended range also allows for integrating optical signal conditioning devices into the measurement setup, facilitating more comprehensive testing of complex optical assemblies. This makes the SPA-110 an ideal choice for large-scale optical testing environments, including:
● Silicon Photonics Testing: Enables detailed analysis of silicon photonic circuits, ensuring device performance and reliability.
● Fiber Optic Component Characterization: Assists in evaluating the performance of various fiber optic components, such as connectors and splices, over extended lengths.
● Compact Fiber Optic Assembly Analysis: Facilitates the examination of compact assemblies, ensuring integrity and performance across the entire system.
The SPA-110’s high spatial resolution and extended measurement range make it versatile for various optical testing applications.
Santec's Swept Photonics Analyzer
Applications of SPA Series
Insertion and Return Loss Measurements
Insertion loss (IL) and return loss (RL) characterization are critical for assessing the performance and efficiency of optical components, particularly in photonic integrated circuits (PICs) and fiber optic devices. The SPA series ensures precise IL and RL measurements by leveraging Optical Frequency Domain Reflectometry (OFDR) technology to analyze the spatial distribution of back reflection and transmission characteristics in optical components.
Key Features Contributing to High-Accuracy IL/RL Measurements
• High Dynamic Range: With a dynamic range exceeding 80 dB, the SPA series can detect weak reflection events, ensuring a comprehensive performance analysis of an optical component.
• Wide Wavelength Sweeping Range: The ability to sweep across a broad wavelength range allows for the detailed characterization of wavelength-dependent loss, verifying that components meet specific performance criteria.
• Attenuation Measurement of PICs: Beyond IL/RL, the SPA series can also measure the total attenuation of a photonic integrated circuit, providing deeper insights into its efficiency and design integrity.
Role of the SPA Series in Quality Assurance and Compliance
The SPA series is valuable for component verification and assessing whether fabricated PICs match the original design specifications. This ensures that no material or structural deviations occur during manufacturing.
• Defect Identification: The system can detect imperfections, such as microbends, misalignments, or material inconsistencies, that could lead to excessive IL or RL.
• Design Validation: It can verify if the fabricated PIC matches the intended design, helping manufacturers identify deviations early in production.
• Group Index Measurement: The SPA series can analyze the group index of the device, determining if the materials used exhibit the correct optical properties and ensuring that no damage occurs during fabrication.
By delivering precise IL, RL, and attenuation measurements, the SPA series plays an integral role in the research, development, and mass production of high-performance optical components.
Analysis of Compact and Complex Devices
The SPA series is designed for high-resolution testing of integrated photonic circuits (PICs) and complex optical assemblies, offering unmatched precision in characterizing intricate structures.
Key Advantages in Testing Complex Optical Assemblies
• High-Resolution Structural Analysis: With spatial resolution better than 5 micrometers, the SPA series can analyze even the smallest features within PICs and silicon photonic devices, ensuring that every element meets the required specifications.
• Comprehensive Device Characterization: The extended measurement range allows for thoroughly evaluating multiple optical components within a single setup, making it ideal for multi-layer PICs and compact optical assemblies.
• Automated and User-Friendly Testing: Advanced software tools simplify device characterization, enabling efficient testing and evaluating highly intricate optical structures.
With its advanced IL/RL characterization, attenuation measurement, and design validation capabilities, the SPA series is a critical tool for manufacturers and researchers, ensuring that optical components meet performance and compliance requirements before deployment.
Conclusion
Optical Frequency Domain Reflectometry (OFDR) and Optical Time Domain Reflectometry (OTDR) serve distinct roles in optical diagnostics. OFDR excels in high-resolution, short-range applications, making it ideal for photonic integrated circuits (PICs), fiber optic sensing, and semiconductor testing. In contrast, OTDR remains the industry standard for long-distance fault detection, particularly in telecommunications and large-scale fiber optic networks.
Santec’s innovations in OFDR technology, mainly through its SPA-110 series, have redefined optical characterization by offering unparalleled spatial resolution, precision IL/RL measurements, and attenuation analysis of PICs. These advancements enable comprehensive testing and quality assessment in research and production environments.
Future developments in high-speed tunable lasers, AI-driven data processing, and advanced proximity sensing will further enhance OFDR’s role in automated optical testing and photonic device characterization. As optical networks and integrated photonics evolve, OFDR will remain a cornerstone technology for next-generation optical diagnostics and high-precision manufacturing.
FAQs
What Is Reflectometry?
Reflectometry is a scientific measurement technique for analyzing wave reflections in different mediums. It involves sending a controlled wave signal into a material or structure and analyzing how it is reflected back. The characteristics of the reflected signal provide valuable information about the material’s composition, discontinuities, or structural defects.
This method plays a significant role in non-destructive testing, telecommunications, and material science. It helps detect hidden irregularities such as cracks, impedance mismatches, or unwanted alterations in a system. Engineers and researchers use reflectometry to evaluate the integrity of optical fibers, pipelines, circuit boards, and materials without physically altering them.
Reflectometry can work with different types of waves, each suited for specific applications:
● Electromagnetic and Optical Waves: These waves are widely used in fiber optics, telecommunications, and electrical testing. In optical applications, reflectometry helps assess fiber integrity, detect defects, and locate signal loss over long distances. In electrical testing, it is used to identify impedance mismatches and discontinuities in conductive paths.
● Acoustic Waves: Primarily applied in medical imaging and geological surveys, acoustic reflectometry measures sound waves reflecting off surfaces to detect voids, inconsistencies, or material changes within structures.
Reflectometry ensures accurate diagnostics in applications where precision is crucial. Analyzing wave reflections allows industries to prevent failures, improve system performance, and maintain quality standards in infrastructure and manufacturing.
What is Frequency-Domain Reflectometry (FDR)?
Frequency-domain reflectometry (FDR) is a diagnostic technique that analyzes wave reflections by varying signal frequencies. Unlike time-domain reflectometry, which sends short pulses and measures their return time, FDR works by transmitting continuous wave signals at different frequencies and analyzing how they interact with the system under test.
The process begins with injecting a frequency-swept signal into a medium. As this signal encounters changes in impedance, part of it reflects back. A frequency domain reflectometer collects and interprets these reflections using mathematical transformations, such as the inverse Fast Fourier Transform (IFFT), to derive meaningful spatial and material insights.
One of FDR’s significant advantages is its ability to provide high-resolution short-range analysis. This makes it ideal for applications requiring precise diagnostics, such as:
● Component testing: Evaluating optical and electrical components for defects.
● Optical fiber monitoring: Identifying strain, temperature variations, or microbends in fiber optics.
● Material characterization: Analyzing the dielectric properties of thin films and composite materials.
Compared to time-domain reflectometry, FDR provides enhanced sensitivity in short-range environments, making it particularly useful in controlled lab settings and highly detailed fault detection applications.
What is Time-Domain Reflectometry (TDR)?
Time-domain reflectometry (TDR) operates by sending a short-duration electrical or optical pulse into a system and measuring how long it takes for the signal to return after encountering discontinuities. The delay time of the reflection is directly correlated with the location of impedance changes, allowing for pinpoint fault detection over long distances.
This technique is commonly used in:
● Cable testing: Detecting breaks, short circuits, and impedance mismatches in electrical wiring and transmission lines.
● Telecommunications: Locating fiber optic faults in large-scale network infrastructures.
● Geophysical applications: Assessing soil moisture and underground material characteristics.
TDR excels in identifying faults across long distances, making it the preferred choice for applications where large-scale monitoring is required. However, its resolution is lower compared to frequency-domain reflectometry. While TDR is excellent for broad network diagnostics, FDR is more effective when high precision is needed for small-scale defect analysis.
Both techniques serve unique purposes, and their selection depends on the application’s specific requirements, such as range, accuracy, and environmental conditions.